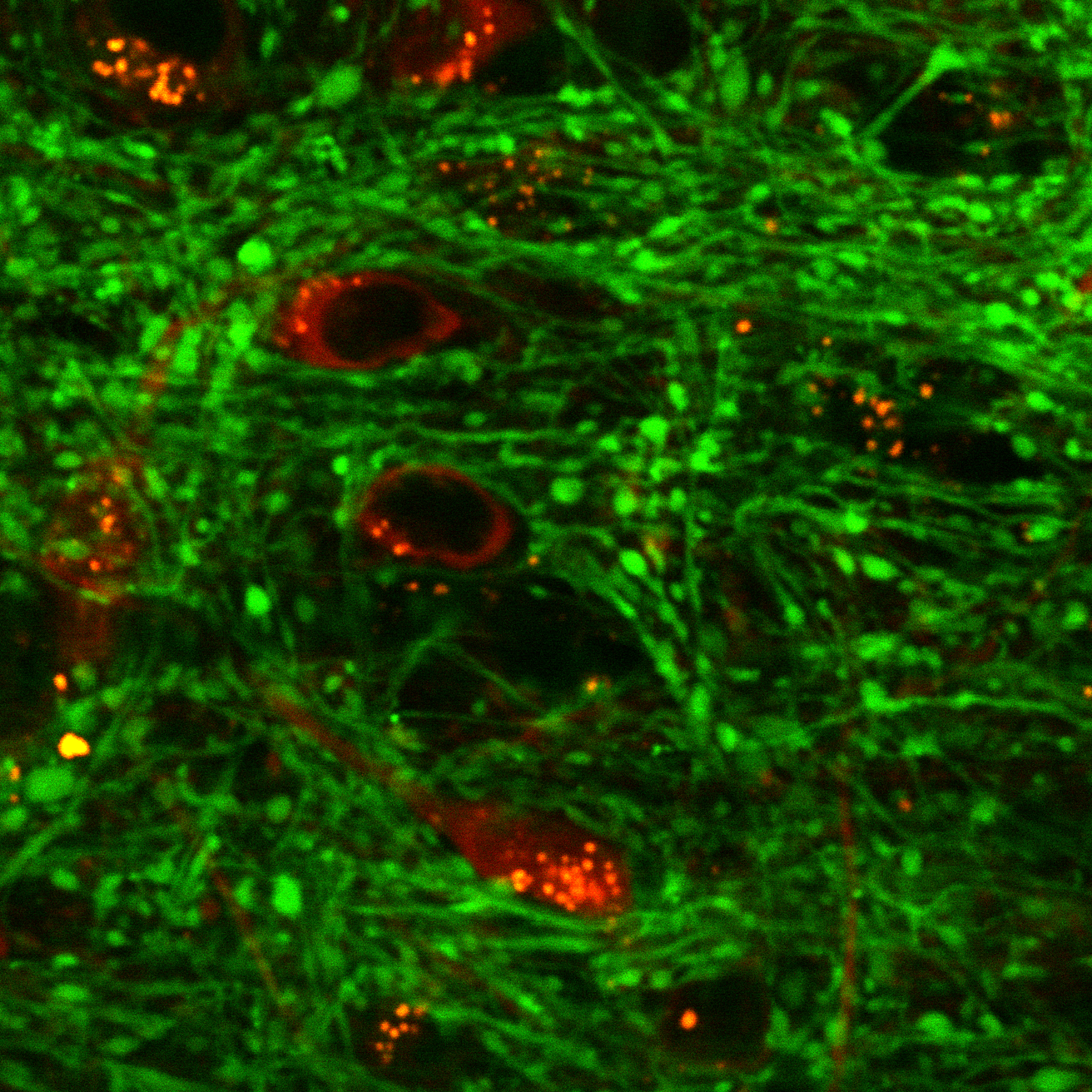
Molecular and Cellular Mechanisms of Pain
Chronic pain management remains one of the most serious public health problems. We use an array of molecular biological and genetic techniques, combined with patch-clamp recordings and fluorescent imaging of intracellular Ca2+, Na+ and pH changes in pain-conducting neurons (called nociceptors), as well as behavioral studies to address two broad sets of questions related to chronic pain pathogenesis. The first set of questions focuses on relatively rapid changes to nociceptor excitability and synaptic transmission that are induced by proinflammatory mediators generated by immune and glial cells at the site of injury or inflammation, and mediated via phosphorylation of so-called pain channels, including TRPV1, TRPA1 and voltage-gated Na+ channels Nav1.7, 1.8 and 1.9. We are particularly interested in the role of the complement system factors C3a and C5a in regulating nociceptor excitability and function. The second set of questions examines the long-term changes to the nociceptor molecular composition and function in response to injury or inflammation, and involves alterations in gene expression. We particularly focus on the Ca2+-dependent transcription factor NFAT that regulates expression of a number of proteins implicated in pain sensitization, such as COX-2, BDNF, chemokine receptor CCR2 and several voltage-gated K+ channels.
Function and Regulation of Neuronal Mitochondria in Health and Disease
Another line of investigation focuses on the function of mitochondria in regulating neuronal plasticity and survival under conditions of stress or illness, such as hyperglycemia in diabetes or exposure to toxic concentrations of glutamate following ischemic stroke. In addition to generating energy, mitochondria play critical role in neuronal signaling, and particularly, in the regulation of Ca2+ homeostasis and Ca2+-dependent processes. Impaired mitochondrial Ca2+ regulation contributes to neuronal damage in stroke and neurodegenerative disorders. Mitochondria are highly dynamic organelles that can rapidly undergo fission and fusion, and the mitochondrial fission and fusion (MFF) balance can significantly impact dendritic and axonal morphogenesis, synaptic plasticity and neuronal survival. We use a multidisciplinary approach (electrophysiology, confocal microscopy, brain slice recordings, animal models of diabetes and stroke) to better understand regulatory mechanisms and function of mitochondrial Ca2+ transport in neurons, its dependence on the MFF status, and the role of mitochondrial Ca2+ and MFF in protecting neurons from glutamate toxicity as well as hyperglycemia-induced axonal degeneration.